Fundamentals of Dehydrating a Refrigerant System
The two most frequent questions service technicians ask about dehydration are:
What size vacuum pump should be used to perform a good refrigeration/air conditioning system dehydration job?
How long should the pump be left on the system to assure removal of all moisture?
To give specific answers to these questions, you need to know the cubic capacity of the system to be dehydrated; amount of moisture both visible and invisible present in the system; I.D. and length of the connecting line as well as the restrictions within the system itself (cap tubes, valves, etc.) which might cause back pressures.
Rather than supplying fast, pat answers to these important questions and then trying to justify them, let's start by covering the basic fundamentals of dehydrating a refrigerant system.
Moisture In A Refrigerant System
While it is important to realize that moisture in a refrigerant system is the underlying cause of most problems and complaints, it is equally important to learn why.
Basically, moisture can be classified as visible and invisible. Occasionally, liquid water is found in systems, but this is unusual. Invisible moisture, or water vapor, is the culprit which causes the greatest trouble in refrigeration and air conditioning systems.
A single drop of water may look harmless, but to a refrigerant system, it is a monster, the number one enemy of service technicians. What makes it so formidable is the fact that moisture enters a system easily and is hard to remove. Here is what it does to a system:
First, it creates "freeze-ups." Moisture will be picked up by the refrigerant and be transported through refrigerant lines in a fine mist which forms ice crystals at the point of expansion (expansion valve).
Ice crystals retard or stop the flow of the refrigerant, causing loss of cooling. As the expansion valve warms, due to the lack of refrigerant, the ice melts and passes through the expansion valve. The refrigerant will then start again until the moisture returns to the expansion valve and once more builds ice crystals. The result is intermittent cooling.
Whether a "freeze-up" actually occurs depends primarily upon the amount of water and the size of the ice particles formed. But a "freeze-up" is not the only problem caused by moisture. It can also cause corrosion, which can present serious trouble.
Moisture in the form of water can cause corrosion after a period of time. However, moisture mixed with refrigerant creates much more corrosion trouble. Refrigerant such as R-12, containing chlorine, will slowly hydrolyze with water and form hydrochloric acids. This acid greatly increases the corrosion of metals and could corrode copper plating.
Heat increases the rate of corrosion due to acids because higher temperatures accelerate the acid-forming process. This acid attacks all the materials it contacts.
Refrigerant oil presents another problem caused by moisture. Refrigerant oil is an exception to the rule that "oil and water don't mix." In fact, refrigerant oil attracts moisture and will absorb it rapidly if left open to the atmosphere. Water-formed acid mixes with refrigerant oil, forming a closely bonded mixture of fine globules. The effect is called "sludging" and greatly reduces the oil's lubricating ability.
Corrosion becomes troublesome from the operating standpoint when metallic surfaces are eaten away and a solid, detachable product is formed. This formation is also known as a "sludge." Sludge can cause a variety of problems. It will plug fine strainers, expansion valves and capillary tubes. And because it usually contains acids, sludge corrodes whatever it clings to, accelerating system damage.
The most effective way to eliminate moisture from a system is with a good, high vacuum pump.
Effects Of Pressure and Temperature on the Boiling Points of Water
A high vacuum pump is capable of removing all moisture from a hermetic system by reducing internal system pressures to the boiling point of water at normal temperatures. For those being introduced here to high vacuum work, it should be stated that a vacuum pump does not "suck out" the liquid moisture, but rather causes it to boil in to a vapor state which can be harmlessly removed from the system and exhausted through the vacuum pump.
The planet Earth is surrounded by matter in a gaseous state composed of about 78% nitrogen, 31% oxygen and 1% a mix of rare gases. Together they form our atmosphere, which extends approximately 600 miles above the earth and is held to the earth by gravity. Being a gas, the atmosphere has weight, and that weight is measured, as in any fluid whether liquid or gas, in pounds per square inch (psi).
If you were to take a square inch column of the air extending six hundred miles above the earth, its weight and pressure exerted on the earth at sea level would be 14.7 lbs. This is called atmospheric pressure . Any pressure above atmospheric pressure is referred to as gauge pressure. Pressures below are referred to as vacuum.
This same square inch column of air exerting 14.7 psi can support a one-inch square column of mercury (Hg) 29.92 inches high. This concept can best be understood by comparing it to a teeter-totter.
When a one square inch column of Hg 29.92" high is placed on one end of the teeter-totter, and a 14.7 lb weight on the other end, the board will be balanced.
Atmospheric pressure decreases at higher elevations. As stated, 600 miles of atmosphere at sea level is equivalent to 14.7 psi and/or 29.92 inch column of mercury (Hg). Going above sea level, to the summit of Mt. Whitney, for example, eliminates some of the 600 miles of atmosphere and, consequently, some of the pressure.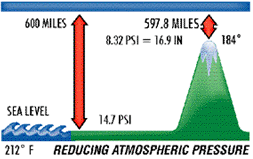
Atmospheric pressure, therefore, governs the boiling point of water. At sea level, where atmospheric pressure is 14.7 psi (29.92" Hg), water boils at 212° F, but on Mt. Whitney, where atmospheric pressure is 8.32 psi (16.9: Hg), water boils at 184° F. The lower the atmospheric pressure is, the lower the boiling point of water. Therefore, if we can significantly reduce the atmospheric pressure inside a sealed refrigerant system, we can vaporize (or boil) moisture at even –90° F. This principle is illustrated in the chart below.
- Transport the system to a higher elevation where the ambient temperature is sufficient to boil water at the existing psi.
- Apply heat to the system causing the moisture to boil.
- Employ a high vacuum pump to reduce the pressure and boiling point of water.
The first two choices are impractical. Thus, a high vacuum pump is an essential aid to every service technician.
High Vacuum/Deep Vacuum
As illustrated above, the purpose of a vacuum pump is to reduce the internal system pressure of a refrigeration/air conditioning system so moisture and other contaminants can be removed.
The term "high vacuum" describes a condition where the internal system pressure is extremely low, or close to a perfect vacuum. The higher the vacuum is in a system, the closer the micron reading is to 0 microns. Deep vacuum can be thought of in the same way. The deeper a vacuum is, the closer the micron reading is to 0 microns.
"High vacuum" and "deep vacuum" describe the same condition inside a closed system. For refrigeration/air conditioning service applications, high vacuum = good vacuum, or a low micron reading on the system.
Selecting High Vacuum Pumps
To make the following discussion easier to understand, it would be best from this point on to think of pressure (psi) as the amount of mercury it will support, e.g. atmospheric pressure at 29.92" Hg instead of 14.7 psi. This will permit us to use Chart II as a visual aid when determining the vacuum which must be attained to boil water under various ambient temperatures.
Chart II shows a vacuum pump capable of eliminating all but one inch of mercury. It is able to remove moisture at an ambient temperature of 80 degrees F or over. While any pump pulling within one inch of atmospheric pressure can eliminate moisture, it must also be capable of holding that vacuum throughout the dehydration process. In addition, it must pull that vacuum on the entire system and not simply at the intake of the pump.
Gas Ballast (Vented Exhaust) Pumps
The gas ballast or vented exhaust feature on some vacuum pumps permits relatively dry air from the atmosphere to enter the second stage of the pump. This air reduces the compression in the final stage, which helps to prevent moisture from condensing into a liquid and mixing with the vacuum pump oil. Here's a comparison to show how it works:
Imagine a damp towel being twisted until water drops out. This can be compared to a high vacuum pump which is not equipped with a gas ballast. Moisture being pulled from a wet refrigerant system is compressed internally in the vacuum pump and condenses into a liquid. Now imagine the same damp towel entwined with a dry towel and then twisted. It would take a considerable amount of twisting before any water would drop out. Thus the process of the gas ballast arrangement permits the moisture-laden air passing through the pump to mix with relatively dry air to such a degree that compression does not cause condensation.
The damp towel comparison also illustrates why this valving feature cannot handle large amounts of moisture. If the towel is almost saturated with water, even the introduction of the completely dry towel will not prevent some of the water from dropping out when the two are compressed. Because of this, some pumps are designed to run with both high internal temperatures (to reduce moisture condensation in the oil) and a gas ballast.
Due to the "severe" application of a vacuum pump when used to "boil water," it is necessary to select a quality two-stage model equipped with a gas ballast and high internal running temperature to achieve adequate performance over a long period of time. However, even the best pump must have regular maintenance to perform optimally.
Frequent oil changes should be anticipated and considered as the single most important factor in a preventative maintenance program. Remember, even a pump equipped with a gas ballast cannot handle large amounts of moisture without some being condensed into the oil. If allowed to remain inside the pump, this moisture will result in lock ups or loss of efficiency and/or capacity. Normally, oil changes will not be required during a single dehydration job. But it would be well to change oil after each major pump down. This is especially critical when pumping down a system known to be wet or experiencing a compressor burn-out.
Factors Affecting The Speed At Which A Pump Can Dehydrate A Refrigerant System
Several factors influence the "pumping speed" of a high vacuum pump, and thus the time required to remove all moisture from a refrigerant system. Some of the most important are: the cubic feet of the system; the amount of moisture contained within the system; the ambient temperature present; internal restrictions within the system; external restrictions between the system and the vacuum source; and the size of the pump.
The only factors under the control of the service technician are the external restrictions between the system and the vacuum pump.
Let us assume that all other variable factors affecting pump down time are equal within a system. Variable pressures within a system attempt to equalize one another. The higher pressure "flows" toward the lower pressure. The above illustration shows the variable pressure creating a vacuum of 100 microns and the system at higher atmospheric pressure. The higher pressure in the system will flow toward the vacuum pump until it is reduced or equal to the 100 microns of pressure. The speed at which it will flow is controlled by the I.D. and length of the connecting line. Laboratory tests show that pump down time can be significantly reduced by use of larger diameter hoses. For optimum pumping speed, keep access lines as short in length and as large in diameter as possible.
All of us have released an inflated balloon, allowing the escaping air pressure to propel it on a wild course. At other times you have inflated a balloon and stretched its stem, allowing the air to escape with a squealing sound. In effect, you were controlling the connecting I.D. as in the above illustration and, in turn, controlling the time it took to release all of its pressure. The principal is identical — the higher pressure is trying to move toward the lower pressure. These illustrations stress the importance of eliminating all external restrictions whenever possible.
In answer to our first question, it is perfectly acceptable to use a 4 CFM or larger vacuum pump on a small system. Using too small of a pump on a large system, e.g. a 1.2 CFM vacuum pump on a 40 ton unit, could cause the pump to operate in a "free air" condition for an extended period of time, thus ri sking premature pump wear.
To answer our second question, the best way to know for sure that the system has been thoroughly dehydrated is by using a thermistor vacuum gauge. This prevents wasting time by pulling down after evacuation is complete or risking inadequate dehydration.
When reading vacuum, remember that the location of the gauge tube will affect the reading. The closer the gauge is to the vacuum pump, the lower the reading will be. To get the most reliable reading, isolate the vacuum pump using a vacuum valve assembly. Let the pressure to equalize throughout the system, then take a final reading.
Robinair offers a complete line of thermistor vacuum gauges. Our model 14010 indicates the vacuum level from 25,000 to 0 microns. Model 14830A shows vacuum level in 10 segments from 25,000 to 50 microns. The pocket-sized Model 14777 measures in ever smaller segments from 20,000 to 50 microns. To save service time, look for a thermistor vacuum gauge that fits the kind of service work you do and use it on a regular basis.
|